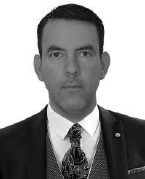
Scientia et Technica Año XXVIII, Vol. 28, No. 01, enero-marzo de 2023. Universidad Tecnológica de Pereira.
Subscripts
a absorbed
p constant pressure
T Total
VI.
ACKNOWLEDGMENT
We are grateful to the directors of the Unidades Tecnológicas
de Santander, especially the Research and Extension
Directorate
REFERENCES
[1] D. Kizildag, J. Castro, H. Kessentini, E. Schillaci, and J. Rigola,
“First test field performance of highly efficient flat plate solar
collectors with transparent insulation and low-cost overheating
protection,” Sol. Energy, vol. 236, no. February, pp. 239–248, 2022.
DOI: 10.1016/j.solener.2022.02.007.
[2] B. V. Balakin, M. Stava, and A. Kosinska, “Photothermal convection
of a magnetic nanofluid in a direct absorption solar collector,” Sol.
Energy, vol. 239, no. April, pp. 33–39, 2022. DOI:
10.1016/j.solener.2022.04.027.
[3] W. Ajbar, J. A. Hernández, A. Parrales, and L. Torres, “Thermal
efficiency improvement of parabolic trough solar collector using
different kinds of hybrid nanofluids,” Case Stud. Therm. Eng., vol.
42, no. November 2022, p. 102759, 2023. DOI:
10.1016/j.csite.2023.102759.
[4] D. García-Menéndez, J. C. Ríos-Fernández, A. M. Blanco-Marigorta,
and M. J. Suárez-López, “Dynamic simulation and exergetic analysis
of a solar thermal collector installation,” Alexandria Eng. J., vol. 61,
no. 2, pp. 1665–1677, 2022. DOI: 10.1016/j.aej.2021.06.075.
[5] A. A. Al-Tabbakh, “Numerical transient modeling of a flat plate solar
collector,” Results Eng., vol. 15, no. August, p. 100580, 2022, DOI:
10.1016/j.rineng.2022.100580.
[6] D. De Maio, C. D’Alessandro, A. Caldarelli, M. Musto, and R. Russo,
“Solar selective coatings for evacuated flat plate collectors:
Optimisation and efficiency robustness analysis,” Sol. Energy Mater.
Sol. Cells, vol. 242, no. February, p. 111749, 2022. DOI:
10.1016/j.solmat.2022.111749.
[7] A. Dhaundiyal and D. Atsu, “The effect of thermo-fluid properties of
air on the solar collector system,” Alexandria Eng. J., vol. 61, no. 4,
pp. 2825–2839, 2022. DOI: 10.1016/j.aej.2021.08.015.
[8] K. N. Yehualashet, O. Fatoba, and S. M. Asfaw, “Experimental study
and numerical analysis of thermal performance of corrugated plate
solar collector,” Mater. Today Proc., vol. 62, pp. 2849–2856. 2022,
DOI: 10.1016/j.matpr.2022.02.414.
[9] A. O. Al-Sulttani et al., “Thermal effectiveness of solar collector
using Graphene nanostructures suspended in ethylene glycol–water
mixtures,” Energy Reports, vol. 8, pp. 1867–1882, 2022. DOI:
10.1016/j.egyr.2022.01.007.
[10] A. M. Ajeena, P. Víg, and I. Farkas, “A comprehensive analysis of
nanofluids and their practical applications for flat plate solar
collectors: Fundamentals, thermophysical properties, stability, and
difficulties,” Energy Reports, vol. 8, pp. 4461–4490, 2022. DOI:
10.1016/j.egyr.2022.03.088.
[11] O. Panagopoulos, A. A. Argiriou, A. Dokouzis, S. O. Alexopoulos,
and J. Göttsche, “Optical and thermal performance simulation of a
micro-mirror solar collector,” Energy Reports, vol. 8, pp. 6624–6632,
2022. DOI: 10.1016/j.egyr.2022.05.007.
[12] L. Xu, A. Khalifeh, A. Khandakar, and B. Vaferi, “Numerical
investigating the effect of Al2O3-water nanofluids on the thermal
efficiency of flat plate solar collectors,” Energy Reports, vol. 8, pp.
6530–6542, 2022. DOI: 10.1016/j.egyr.2022.05.012.
[13] E. Gaudino, M. Musto, A. Caldarelli, D. De Luca, E. Di Gennaro, and
R. Russo, “Evaluation of the absorber temperature frequency function
valid for evacuated flat plate collectors,” Energy Reports, vol. 8, pp.
1071–1080, 2022. DOI: 10.1016/j.egyr.2022.05.275.
[14] Y. Wenceslas Koholé, F. Cyrille Vincelas Fohagui, and G. Tchuen,
“Flat-plate solar collector thermal performance assessment via
energy, exergy and irreversibility analysis,” Energy Convers. Manag.
X, vol. 15, no. June, 2022. DOI: 10.1016/j.ecmx.2022.100247.
[15] A. Al-Manea, R. Al-Rbaihat, H. T. Kadhim, A. Alahmer, T. Yusaf,
and K. Egab, “Experimental and numerical study to develop
TRANSYS model for an active flat plate solar collector with an
internally serpentine tube receiver,” Int. J. Thermofluids, vol. 15, no.
August, p. 100189, 2022. DOI: 10.1016/j.ijft.2022.100189.
[16] T. desisa Rago, “Experimental and Numerical Investigation of Heat
Transfer Characteristics in Solar Flat Plate Collector Using
Nanofluids,” SSRN Electron. J., vol. 18, no. March, p. 100325, 2022.
DOI: 10.2139/ssrn.4282071.
[17] P. Pourmoghadam, M. Farighi, F. Pourfayaz, and A. Kasaeian,
“Annual transient analysis of energetic, exergetic, and economic
performances of solar cascade organic Rankine cycles integrated with
PCM-based thermal energy storage systems,” Case Stud. Therm.
Eng., vol. 28, p. 101388, 2021. DOI: 10.1016/j.csite.2021.101388.
[18] K. Lentswe, A. Mawire, P. Owusu, and A. Shobo, “A review of
parabolic solar cookers with thermal energy storage,” Heliyon, vol. 7,
no. 10, p. e08226, 2021. DOI: 10.1016/j.heliyon.2021.e08226.
[19] G. Sadeghi, M. Najafzadeh, and H. Safarzadeh, “Utilizing Gene-
Expression Programming in Modelling the Thermal Performance of
Evacuated Tube Solar Collectors,” J. Energy Storage, vol. 30, no.
March 2020, p. 101546, 2020, DOI: 10.1016/j.est.2020.101546.
[20] J. Deng, T. S. O’Donovan, Z. Tian, J. King, and S. Speake, “Thermal
performance predictions and tests of a novel type of flat plate solar
thermal collectors by integrating with a freeze tolerance solution,”
Energy Convers. Manag., vol. 198, no. April, p. 111784, 2019, DOI:
10.1016/j.enconman.2019.111784.
[21] V. Unterberger, K. Lichtenegger, V. Kaisermayer, M. Gölles, and M.
Horn, “An adaptive short-term forecasting method for the energy
yield of flat-plate solar collector systems,” Appl. Energy, vol. 293, p.
116891, 2021. DOI: 10.1016/j.apenergy.2021.116891.
[22] W. Villasmil, M. Troxler, R. Hendry, P. Schuetz, and J. Worlitschek,
“Control strategies of solar heating systems coupled with seasonal
thermal energy storage in self-sufficient buildings,” J. Energy
Storage, vol. 42, 2021. DOI: 10.1016/j.est.2021.103069.
[23] M. M. Ali, O. K. Ahmed, and E. F. Abbas, “Performance of solar
pond integrated with photovoltaic/thermal collectors,” Energy
Reports, vol. 6, pp. 3200–3211, 2020. DOI:
10.1016/j.egyr.2020.11.037.
[24] T. Zhang, G. Lu, X. Zhai, and B. Li, “Structure optimization of a
phase change material integrated solar air collector/storage unit based
upon phase change analysis,” Energy Reports, vol. 7, pp. 1828–1836,
2021, DOI: 10.1016/j.egyr.2021.03.040.
Arly Darío Rincón Quintero was born
in Aguachica, Cesar, Colombia in 1982.
He received the degree in mechanical
engineering from Francisco de Paula
Santander University, Colombia, in 2005
and the degree Master in Energy
Efficiency and Sustainability from the
University of the Basque Country
UPV/EHU, Bilbao, España, in 2013. He is currently pursuing
the Ph.D. degree in Energy efficiency and sustainability in
engineering and architecture with Basque Country
UPV/EHU,Bilbao, España. He is a senior researcher before
MinCiencias, Colombia associate professor at the Unidades
Tecnologicas de Santander, in the Faculty of Natural Sciences
and Engineering.
ORCID: https://orcid.org/0000-0002- 4479-5613